What are the safest and cleanest sources of energy?
Fossil fuels are the dirtiest and most dangerous energy sources, while nuclear and modern renewable energy sources are vastly safer and cleaner.
Summary
All energy sources have negative effects, but they differ enormously in size: as we will see, fossil fuels are the dirtiest and most dangerous, while nuclear and modern renewable energy sources are vastly safer and cleaner. From the perspectives of both human health and climate change, it matters less whether we transition to nuclear power or renewable energy and more that we stop relying on fossil fuels.
Energy has been critical to human progress over the last few centuries. As the United Nations rightly says: “energy is central to nearly every major challenge and opportunity the world faces today.”
But while energy brings us massive benefits, it’s not without its downsides. Energy production can have negative impacts on human health and the environment in three ways.
The first is air pollution: millions of people die prematurely every year as a result of air pollution. Fossil fuels and the burning of biomass — wood, dung, and charcoal — are responsible for most of those deaths.
The second is accidents. This includes accidents in the mining and extraction of fuels — coal, uranium, rare metals, oil, and gas. It also includes accidents in transporting raw materials and infrastructure, the construction of the power plant, or its maintenance.
The third is greenhouse gas emissions: fossil fuels are the main source of greenhouse gases, the primary driver of climate change. In 2020, 91% of global CO2 emissions came from fossil fuels and industry.1
No energy source is completely safe. All have short-term impacts on human health, either through air pollution or accidents, and they all have long-term impacts by contributing to climate change.
But, their contribution to each differs enormously. Fossil fuels are both the dirtiest and most dangerous in the short term and emit the most greenhouse gases per unit of energy. This means that there are thankfully no trade-offs here: low-carbon energy sources are also the safest. From the perspective of both human health and climate change, it matters less whether we transition to nuclear power or renewable energy and more that we stop relying on fossil fuels.
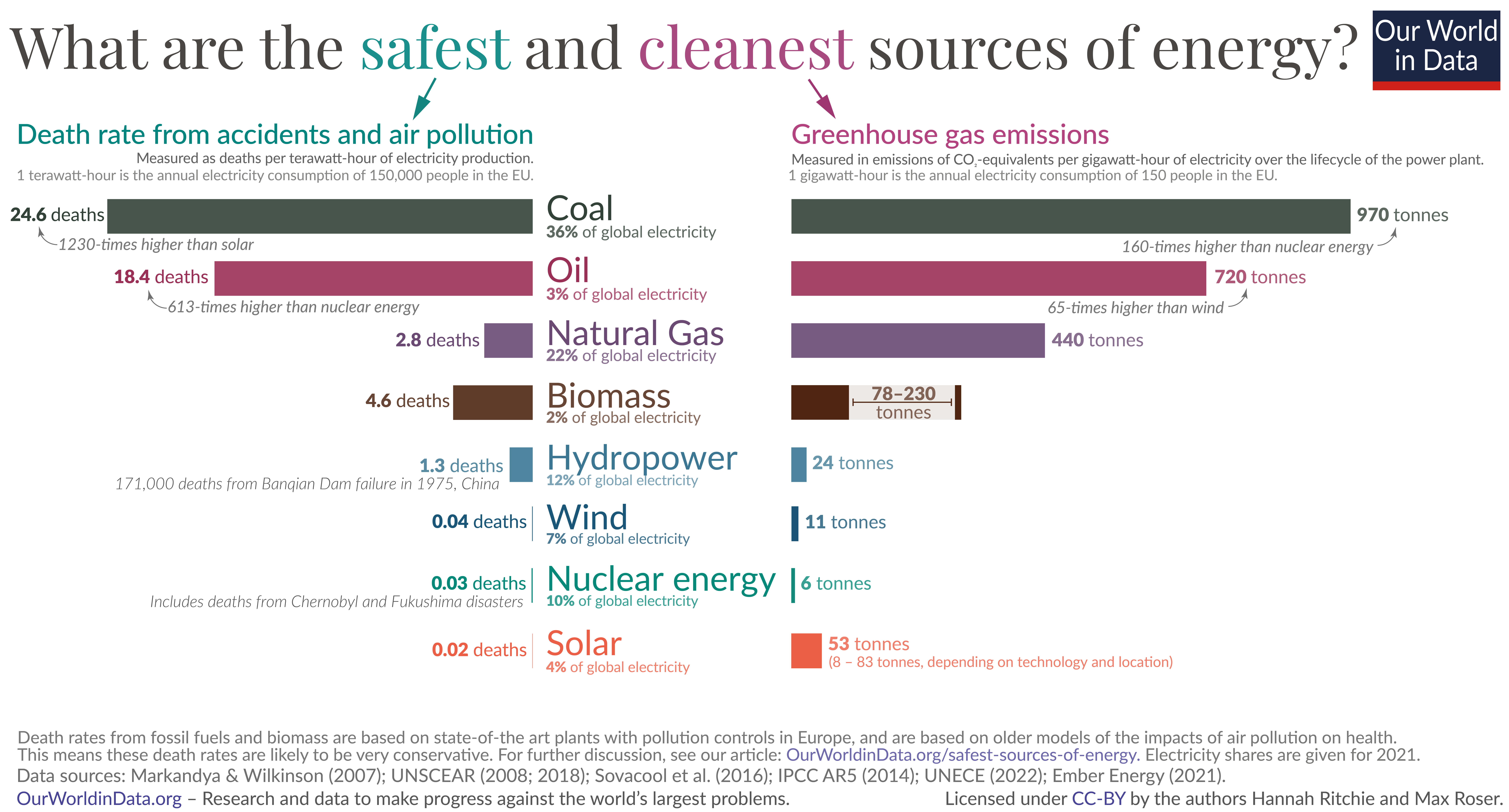
Nuclear and renewables are far, far safer than fossil fuels
Before we consider the long-term impacts of climate change, let’s look at how each source stacks up in terms of short-term health risks.
To make these comparisons fair, we can’t just look at the total deaths from each source: fossil fuels still dominate our global electricity mix, so we would expect that they would kill more people.
Instead, we compare them based on the estimated number of deaths they cause per unit of electricity. This is measured in terawatt-hours. One terawatt-hour is about the same as the annual electricity consumption of 150,000 citizens in the European Union.2
This includes deaths from air pollution and accidents in the supply chain.3
Let’s look at this comparison in the chart. Fossil fuels and biomass kill many more people than nuclear and modern renewables per unit of electricity. Coal is, by far, the dirtiest.
Even then, these estimates for fossil fuels are likely to be very conservative. They are based on power plants in Europe, which have good pollution controls and are based on older models of the health impacts of air pollution. As I discuss in more detail at the end of this article, global death rates from fossil fuels based on the most recent research on air pollution are likely to be even higher.
Our perceptions of the safety of nuclear energy are strongly influenced by two accidents: Chernobyl in Ukraine in 1986 and Fukushima in Japan in 2011. These were tragic events. However, compared to the millions that die from fossil fuels every year, the final death tolls were very low. To calculate the death rates used here, I assume a death toll of 433 from Chernobyl, and 2,314 from Fukushima.4 If you are interested, I look at how many died in each accident in detail in a related article.
The other source heavily influenced by a few large-scale accidents is hydropower. Its death rate since 1965 is 1.3 deaths per TWh. This rate is almost completely dominated by one event: the Banqiao Dam Failure in China in 1975, which killed approximately 171,000 people. Otherwise, hydropower was very safe, with a death rate of just 0.04 deaths per TWh — comparable to nuclear, solar, and wind.
Finally, we have solar and wind. The death rates from both of these sources are low but not zero. A small number of people die in accidents in supply chains — ranging from helicopter collisions with turbines, fires during the installation of turbines or panels, and drownings on offshore wind sites.
People often focus on the marginal differences at the bottom of the chart — between nuclear, solar, and wind. This comparison is misguided: the uncertainties around these values mean they are likely to overlap.
The key insight is that they are all much, much safer than fossil fuels.
Nuclear energy, for example, results in 99.9% fewer deaths than brown coal; 99.8% fewer than coal; 99.7% fewer than oil; and 97.6% fewer than gas. Wind and solar are just as safe.
Putting death rates from energy in perspective
Looking at deaths per terawatt-hour can seem abstract. Let’s try to put it in perspective.
Let’s consider how many deaths each source would cause for an average town of 150,000 people in the European Union, which — as I’ve said before — consumes one terawatt-hour of electricity per year. Let’s call this town ‘Euroville’.
If Euroville were completely powered by coal, we’d expect at least 25 people to die prematurely every year from it. Most of these people would die from air pollution.
This is how a coal-powered Euroville would compare with towns powered entirely by each energy source:
- Coal: 25 people would die prematurely every year;
- Oil: 18 people would die prematurely every year;
- Gas: 3 people would die prematurely every year;
- Hydropower: In an average year, 1 person would die;
- Wind: In an average year, nobody would die. A death rate of 0.04 deaths per terawatt-hour means every 25 years, a single person would die;
- Nuclear: In an average year, nobody would die — only every 33 years would someone die.
- Solar: In an average year, nobody would die — only every 50 years would someone die.
The safest energy sources are also the cleanest
The good news is that there is no trade-off between the safest sources of energy in the short term and the least damaging for the climate in the long term. They are one and the same, as the chart below shows.
In the chart on the left-hand side, we have the same comparison of death rates from accidents and air pollution that we just looked at. On the right, we have the amount of greenhouse gases emitted per unit of electricity production.
These are not just the emissions from the burning of fuels but also the mining, transportation, and maintenance over a power plant’s lifetime.5
Again, coal is the dirtiest fuel. It emits much more greenhouse gases than other sources — more than a hundred times more than nuclear.
Oil and gas are also much worse than nuclear and renewables but to a lesser extent than coal.
Unfortunately, the global electricity mix is still dominated by fossil fuels: coal, oil, and gas account for around 60%. If we want to stop climate change, we have a great opportunity in front of us: we can transition away from them to nuclear and renewables and also reduce deaths from accidents and air pollution as a side effect.6
This transition will not only protect future generations, but it will also come with huge health benefits for the current one.
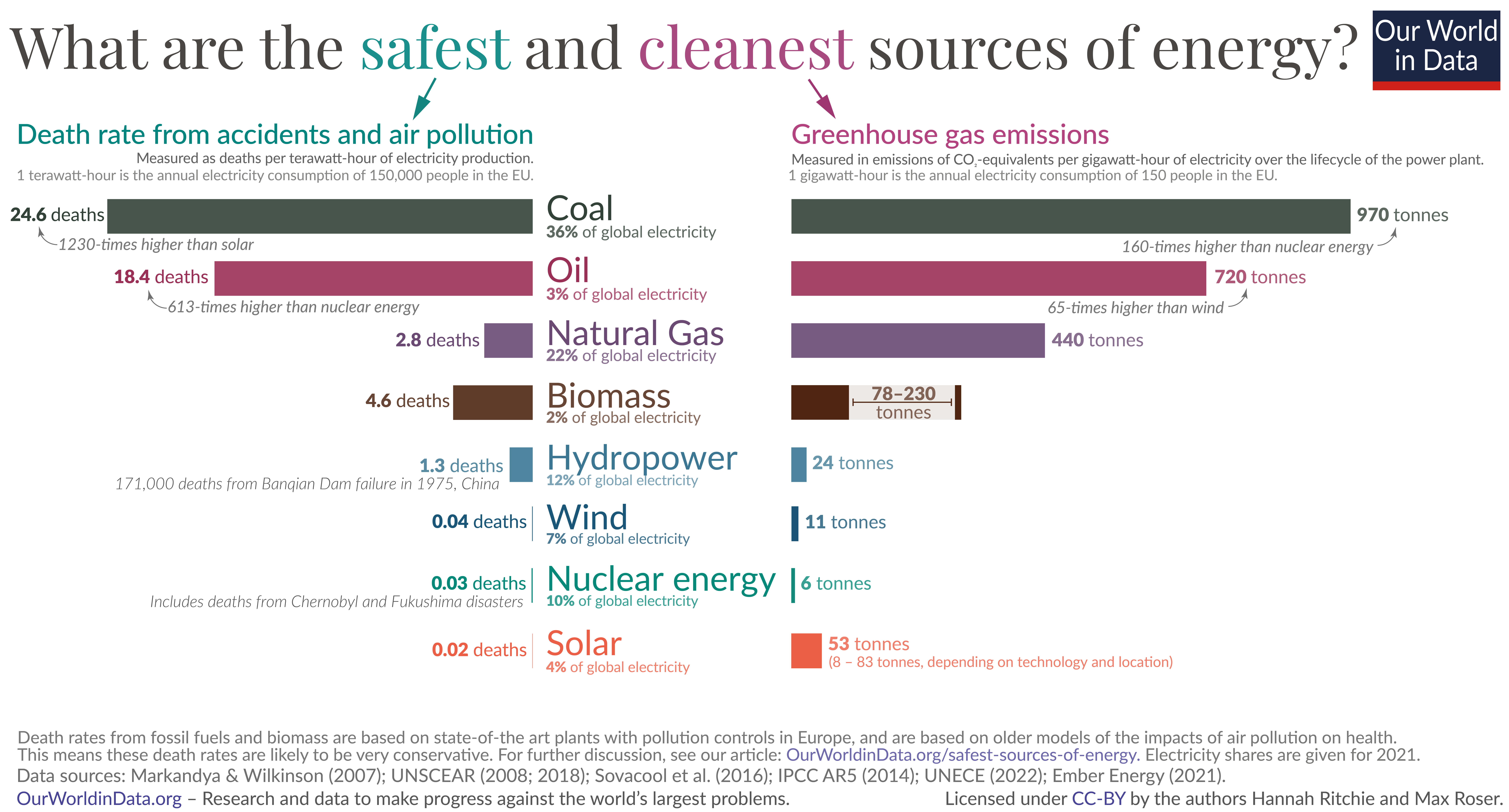
Methodology and notes
Global average death rates from fossil fuels are likely to be even higher than reported in the chart above
The death rates from coal, oil, and gas used in these comparisons are sourced from the paper of Anil Markandya and Paul Wilkinson (2007) in the medical journal, The Lancet. To date, these are the best peer-reviewed references I could find on the death rates from these sources. These rates are based on electricity production in Europe.
However, there are three key reasons why I think that these death rates are likely to be very conservative, and the global average death rates could be substantially higher.
- European fossil fuel plants have strict pollution controls. Power plants in Europe tend to produce less pollution than the global average and much less than plants in many low-to-middle-income countries. This means that the pollution generated per unit of electricity will likely be higher in other parts of the world.
- In other countries, more people will live closer to power plants and be exposed to more pollution. If two countries produce the same amount of coal power and both have the same pollution controls, the country where power plants are closer to urban centers and cities will have a higher death toll per TWh. This is because more people will be exposed to higher levels of pollution.Power plants in countries such as China tend to be located closer to cities in many countries than they are in Europe, so we would expect the death rate to be higher than the European figures found by Markandya and Wilkinson (2007).7
- More recent research on air pollution suggests the health impacts are more severe than earlier research suggested. The analysis by Markandya and Wilkinson was published in 2007. Since then, our understanding of the health impacts of air pollution has increased significantly. More recent research suggests the health impacts are more severe. My colleague, Max Roser, shows this evolution of the research on air pollution deaths in his review of the literature here.Another reason to suspect that the global average rates are much higher is the following: if we take the death rates from Markandya and Wilkinson (2007) and multiply them by global electricity production, the resulting estimates of total global deaths from fossil fuel electricity are much lower than the most recent research.If I multiply the Markandya and Wilkinson (2007) death rates for coal, oil, and gas by their respective global electricity outputs in 2021, I get a total death toll of 280,000 people.8This is much lower than the estimates from more recent research. For example, Leliveld et al. (2018) estimate that 3.6 million die from fossil fuels yearly.9 Vohra et al. (2021) estimate more than double this figure: 8.7 million.10Not all deaths from fossil fuel air pollution are due to electricity production. But we can estimate how many deaths do. In a recent paper, Leliveld and his colleagues estimated the breakdown of air pollution deaths by sector. They estimate that 12% of all air pollution deaths (from fossil fuels and pollution from other sources) come from electricity production.11
By my calculations, we would expect that 1.1 million to 2.55 million people die from fossil fuels used for electricity production each year.12The estimates we get from Markandya and Wilkinson (2007) death rates undercount by a factor of 4 to 9. This would suggest that actual death rates from fossil fuels could be 4 to 9 times higher. That would give a global average death rate from coal of 93 to 224 deaths per TWh.Unfortunately, we do not have more up-to-date death rates for coal, oil, and gas to reference here, but improved estimates are sorely needed. The current death rates shown are likely to be underestimated.
We need a timely global database on accidents in energy supply chains
The figures we reference on accidents from nuclear, solar, and wind are based on the most comprehensive figures we have to date. However, they are imperfect, and no timely dataset tracking these accidents exists. This is a key gap in our understanding of the safety of energy sources — and how their safety changes over time.
To estimate death rates from renewable energy technologies, Sovacool et al. (2016) compiled a database of energy-related accidents across academic databases and news reports. They define an accident as “an unintentional incident or event at an energy facility that led to either one death (or more) or at least $50,000 in property damage,” which is consistent with definitions in the research literature.
This raises several questions about which incidents should and shouldn’t be attributed to a given energy technology. For example, this database included deaths related to an incident in which water from a water tank ruptured during a construction test at a solar factory. It’s not clear whether these supply chain deaths should or shouldn’t be attributed to solar technologies.
The comparability of these incidents across the different energy technologies is, therefore, difficult to assess with high certainty. One additional issue with this analysis by Sovacool et al. (2016) is that its database search was limited to English reports or non-English reports that had been translated. Some of these comparisons could therefore be a slight over- or underestimate. It is, however, unlikely that the position of these technologies would change significantly — renewable and nuclear technologies would consistently come out with a much lower death rate than fossil fuels. Consistent data collection and tracking of incidents across all energy technologies would greatly improve these comparisons.
We need improved estimates of the health impacts of the mining of minerals and materials for all energy sources
The figures presented in this research that I rely on do not include any health impacts from radiation exposure from the mining of metals and minerals used in supply chains.
While we might think that this would only have an impact on nuclear energy, analyses suggest that the carcinogenic toxicity of other sources — including solar, wind, hydropower, coal, and gas are all significantly higher across their supply chains.13
These figures only measure workers' potential exposure to toxic elements. They do not give us estimates of potential death rates, which is why we do not include them in our referenced figures above.
However, the inclusion of these figures would not change the relative results, overall. Fossil fuels — coal, in particular — have a higher carcinogenic toxicity than both nuclear and renewables. Hence the relative difference between them would actually increase, rather than decrease. The key insight would still be the same: fossil fuels are much worse for human health, and both nuclear and modern renewables are similarly safe alternatives.
However, estimates of the health burden of rare minerals in energy supply chains are still an important gap to fill, so that we can learn about their impact and ultimately reduce these risks moving forward.
Update
This article was first published in 2017. It was last updated in July 2022 based on more recent analysis and estimates of energy safety. Figures for the carbon intensity of different electricity sources were updated in March 2024 based on the most recent analysis of lifecycle emissions from the United Nations Economic Commission for Europe (UNECE).
Endnotes
Pierre Friedlingstein, Matthew W. Jones, Michael O'Sullivan, Robbie M. Andrew, Dorothee, C. E. Bakker, Judith Hauck, Corinne Le Quéré, Glen P. Peters, Wouter Peters, Julia Pongratz, Stephen Sitch, Josep G. Canadell, Philippe Ciais, Rob B. Jackson, Simone R. Alin, Peter Anthoni, Nicholas R. Bates, Meike Becker, Nicolas Bellouin, Laurent Bopp, Thi Tuyet Trang Chau, Frédéric Chevallier, Louise P. Chini, Margot Cronin, Kim I. Currie, Bertrand Decharme, Laique M. Djeutchouang, Xinyu Dou, Wiley Evans, Richard A. Feely, Liang Feng, Thomas Gasser, Dennis Gilfillan, Thanos Gkritzalis, Giacomo Grassi, Luke Gregor, Nicolas Gruber, Özgür Gürses, Ian Harris, Richard A. Houghton, George C. Hurtt, Yosuke Iida, Tatiana Ilyina, Ingrid T. Luijkx, Atul Jain, Steve D. Jones, Etsushi Kato, Daniel Kennedy, Kees Klein Goldewijk, Jürgen Knauer, Jan Ivar Korsbakken, Arne Körtzinger, Peter Landschützer, Siv K. Lauvset, Nathalie Lefèvre, Sebastian Lienert, Junjie Liu, Gregg Marland, Patrick C. McGuire, Joe R. Melton, David R. Munro, Julia E.M.S Nabel Shin-Ichiro Nakaoka, Yosuke Niwa, Tsuneo Ono, Denis Pierrot, Benjamin Poulter, Gregor Rehder, Laure Resplandy, Eddy Robertson, Christian Rödenbeck, Thais M Rosan, Jörg Schwinger, Clemens Schwingshackl, Roland Séférian, Adrienne J. Sutton, Colm Sweeney, Toste Tanhua, Pieter P Tans, Hanqin Tian, Bronte Tilbrook, Francesco Tubiello, Guido van der Werf, Nicolas Vuichard, Chisato Wada Rik Wanninkhof, Andrew J. Watson, David Willis, Andrew J. Wiltshire, Wenping Yuan, Chao Yue, Xu Yue, Sönke Zaehle, Jiye Zeng. Global Carbon Budget 2021, Earth Syst. Sci. Data, 2021.
Per capita electricity consumption in the EU-27 in 2021 was around 6,400 kWh.
1 terawatt-hour is equal to 1,000,000,000 kilowatt-hours. So, we get this figure by dividing 1,000,000,000 by 6,400 ≈ 150,000 people.
The following sources were used to calculate these death rates.
Fossil fuels and biomass = these figures are taken directly from Markandya, A., & Wilkinson, P. (2007). Electricity generation and health. The Lancet, 370(9591), 979-990.
Nuclear = I have calculated these figures based on the assumption of 433 deaths from Chernobyl and 2,314 from Fukushima. These figures are based on the most recent estimates from UNSCEAR and the Government of Japan. In a related article, I detail where these figures come from.
I have calculated death rates by dividing this figure by cumulative global electricity production from nuclear from 1965 to 2021, which is 96,876 TWh.
Hydropower = The paper by Sovacool et al. (2016) provides a death rate for hydropower from 1990 to 2013. However, this period excludes some very large hydropower accidents that occurred prior to 1990. I have, therefore, calculated a death rate for hydropower from 1965 to 2021 based on the list of hydropower accidents provided by Sovacool et al. (2016), which extends back to the 1950s. Since this database ends in 2013, I have also included the Saddle Dam accident in Laos in 2018, which killed 71 people.
The total number of deaths from hydropower accidents from 1965 to 2021 was approximately 176,000. Of those, 171,000 were caused by the 1975 Banqian Dam failure in China.
I have calculated death rates by dividing this figure by cumulative global electricity production from hydropower from 1965 to 2021, which is 138,175 TWh.
Solar and wind = these figures are taken directly from Sovacool, B. K., Andersen, R., Sorensen, S., Sorensen, K., Tienda, V., Vainorius, A., … & Bjørn-Thygesen, F. (2016). Balancing safety with sustainability: assessing the risk of accidents for modern low-carbon energy systems. Journal of Cleaner Production, 112, 3952-3965. In this analysis, the authors compiled a database of as many energy-related accidents as possible based on an extensive search of academic databases and news reports and derived death rates for each source from 1990 to 2013. Since this database has not been extended since then, it’s not possible to provide post-2013 death rates.
UNSCEAR (2008). Sources and effects of Ionizing Radiation. UNSCEAR 2008 Report to the General Assembly with Scientific Annexes. Available online.
Report of the United Nations Scientific Committee on the Effects of Atomic Radiation. General Assembly Official Records, Sixty-eighth session, Supplement No. 46. New York: United Nations, Sixtieth session, May 27–31, 2013.
The main figures used in this analysis come from the United Nations Economic Commission for Europe (UNECE) Lifecycle Assessment of Electricity Generation Options, published in 2022.
These figures are similar to those published by the IPCC, and other energy organizations.
Schlömer S., T. Bruckner, L. Fulton, E. Hertwich, A. McKinnon, D. Perczyk, J. Roy, R. Schaeffer, R. Sims, P. Smith, and R. Wiser, 2014: Annex III: Technology-specific cost and performance parameters. In: Climate Change 2014: Mitigation of Climate Change. Contribution of Working Group III to the Fifth Assessment Report of the Intergovernmental Panel on Climate Change [Edenhofer, O., R. Pichs-Madruga, Y. Sokona, E. Farahani, S. Kadner, K. Seyboth, A. Adler, I. Baum, S. Brunner, P. Eickemeier, B. Kriemann, J. Savolainen, S. Schlömer, C. von Stechow, T. Zwickel and J.C. Minx (eds.)]. Cambridge University Press, Cambridge, United Kingdom and New York, NY, USA.
The figures for some technologies — such as solar — vary significantly depending on where they’re manufactured (and the country's electricity mix). Estimates range from around 23 grams CO2 per kWh to 82 grams.
The carbon intensity of these technologies' production is likely to improve over time. The Carbon Brief provides a clear discussion of the significance of more recent lifecycle analyses in detail here.
Since oil is not conventionally used for electricity production, it is not included in the IPCC’s reported figures per kilowatt-hour. Figures for oil have, therefore, been taken from Turconi et al. (2013). It reports emissions in kilograms of CO2eq per megawatt-hour. Emissions factors for all other technologies are consistent with IPCC results. The range it gives for oil is 530–900: I have taken the midpoint estimate (715 kgCO2eq/MWh, or 715 gCO2eq/kWh).
Turconi, R., Boldrin, A., & Astrup, T. (2013). Life cycle assessment (LCA) of electricity generation technologies: Overview, comparability and limitations. Renewable and Sustainable Energy Reviews, 28, 555-565.
Burgherr, P., & Hirschberg, S. (2014). Comparative risk assessment of severe accidents in the energy sector. Energy Policy, 74, S45-S56.
McCombie, C., & Jefferson, M. (2016). Renewable and nuclear electricity: Comparison of environmental impacts. Energy Policy, 96, 758-769.
Hirschberg, S., Bauer, C., Burgherr, P., Cazzoli, E., Heck, T., Spada, M., & Treyer, K. (2016). Health effects of technologies for power generation: Contributions from normal operation, severe accidents and terrorist threat. Reliability Engineering & System Safety, 145, 373-387.
Luderer, G., Pehl, M., Arvesen, A., Gibon, T., Bodirsky, B. L., de Boer, H. S., … & Mima, S. (2019). Environmental co-benefits and adverse side-effects of alternative power sector decarbonization strategies. Nature Communications, 10(1), 1-13.
Hertwich, E. G., Gibon, T., Bouman, E. A., Arvesen, A., Suh, S., Heath, G. A., … & Shi, L. (2015). Integrated life-cycle assessment of electricity-supply scenarios confirms global environmental benefit of low-carbon technologies. Proceedings of the National Academy of Sciences, 112(20), 6277-6282.
Xie, L., Huang, Y., & Qin, P. (2018). Spatial distribution of coal-fired power plants in China. Environment and Development Economics, 23(4), 495-515.
Coal: 24.62 deaths per TWh * 10,042 TWh = 247,000 deathsOil: 18.43 deaths per TWh * 852 TWh = 16,000 deathsGas: 2.82 deaths per TWh * 6,098 TWh = 17,000 deaths.This sums to a total of 280,000 people.
Lelieveld, J., Klingmüller, K., Pozzer, A., Burnett, R. T., Haines, A., & Ramanathan, V. (2019). Effects of fossil fuel and total anthropogenic emission removal on public health and climate. Proceedings of the National Academy of Sciences, 116(15), 7192-7197.
Vohra, K., Vodonos, A., Schwartz, J., Marais, E. A., Sulprizio, M. P., & Mickley, L. J. (2021). Global mortality from outdoor fine particle pollution generated by fossil fuel combustion: Results from GEOS-Chem. Environmental Research, 195, 110754.
Chowdhury, S., Pozzer, A., Haines, A., Klingmueller, K., Münzel, T., Paasonen, P., ... & Lelieveld, J. (2022). Global health burden of ambient PM2.5 and the contribution of anthropogenic black carbon and organic aerosols. Environment International, 159, 107020.
Leliveld et al. (2019) estimate that 8.8 million people die from all sources of air pollution each year. If we multiply this figure by 12%, we get 1.1 million people.Vohra et al. (2021) estimate that the death toll is 2.4 times higher than Leliveld et al. (2019). This would give a figure of 2.55 million deaths [1.1 million * 2.4]
UNECE (2021). Lifecycle Assessment of Electricity Generation Options. United Nations Economic Commission for Europe.
Cite this work
Our articles and data visualizations rely on work from many different people and organizations. When citing this article, please also cite the underlying data sources. This article can be cited as:
Hannah Ritchie (2020) - “What are the safest and cleanest sources of energy?” Published online at OurWorldInData.org. Retrieved from: 'https://ourworldindata.org/safest-sources-of-energy' [Online Resource]
BibTeX citation
@article{owid-safest-sources-of-energy,
author = {Hannah Ritchie},
title = {What are the safest and cleanest sources of energy?},
journal = {Our World in Data},
year = {2020},
note = {https://ourworldindata.org/safest-sources-of-energy}
}
Reuse this work freely
All visualizations, data, and code produced by Our World in Data are completely open access under the Creative Commons BY license. You have the permission to use, distribute, and reproduce these in any medium, provided the source and authors are credited.
The data produced by third parties and made available by Our World in Data is subject to the license terms from the original third-party authors. We will always indicate the original source of the data in our documentation, so you should always check the license of any such third-party data before use and redistribution.
All of our charts can be embedded in any site.